Equalization & Neutralization...
Equalization...
Definition...
Simply a well - mixed vessel with fluctuating input flow rates and / or concentration with fairly constant output flow
rates and/or concentrations. Processes for waste treatment work best with uniform conditions. Shocks to the bioprocesses
in the form of sudden changes in concentrations of nutrients can cause upsets. If the concentrations or flow rates of the
waste vary greatly, dosages for treatment must be constantly be readjusted. Consider sedimentation. If the input flow
increases suddenly, the settling patterns will be upset to lower collection efficiency. Equalization dampens fluctuations.
Flow equalization can improve performance of subsequent steps significantly. Often the rest of the plant can be designed
with smaller equipment (less capital investment) because of this improvement in performance. Equalization allows reactions
in the equalization tank. There may be aeration both to keep the fluid from becoming anaerobic and smelly and to biodegrade
some of the organic compounds present. More important for industrial wastes that can have wide swings in pH is the reaction
of acids with bases because otherwise each would have to be neutralized with costs for equipment and reagents. Early in the
process, usually following the initial step of collecting debris from the input stream from the sewer.
Process Sketches...
Some common ways of incorporating flow equalization are shown in the sketches :
"In - Line and Side - Line Equalization"...
The top method is called in-line. The side-line shown above it has equalization in the surge tank with
overflow when flow rates are high into the equalization tank. This method makes sense when there is a combined sewer
system for both wastewater and storm water. Much of the time the surge tank is adequate. When there are heavy rains,
this overflows into the equalization tank to be proportioned into the main stream over a period of time. The pump shown
in blue is usually several pumps because one may be down for maintenance. The pumps may have constant flow, either off or
on. One pump may have variable speed that is fairly expensive. The system must have some sort of logical control to decide
which pumps are on and to add back the surplus wastewater at rates that keep the rest of the plant operating with nearly
constant conditions. ludges from the various sedimentation units are digested anaerobically to reduce their organic matter
and to improve handling and drying. The supernatant from treated sludge is rich in organic matter and goes back for more
treatment. The equalization step is a good place to add back this supernatant.
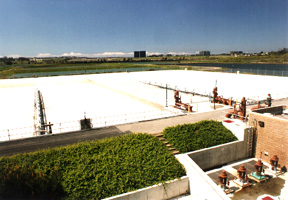
Design of Equalization Units...
Equalization vessels often have sloping sides to keep the head more constant as volume changes.
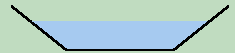
"Sloping Sides"...
A common method for combining aeration and mixing is to have a mixer at the surface to splash the liquid into the air.
Because the level is changing, this mixer must be mounted on floats. The aeration in the equalization vessel will reduce
the BOD of the wastewater by 10 to 20 %. A graphical method is used to specify the volume of an equalization vessel. A plot
of flow rate versus time gets a horizontal line for the average flow rate. All flows above this line are integrated to get
the fluctuating volume for the vessel. This is shown in the next sketch.
"Fluctuating Volume"...
The vessel volume should equal the average flow multiplied by the time period, and then add on the fluctuating volume.
Note that when the flow variation is small, the fluctuating volume is small so that the required vessel size is close to
that based on average flow. When the variation is great, the equalization vessel is quite a bit larger than that based on
the average flow.
Storage Applications...
Storage is considered a necessary control alternative for wet weather flows because of the high volume and variability
associated with stormwater and combined sewer overflows. Some of the most critical problems currently facing wastewater
management agencies are the control of Infiltration/Inflow (I/I)-induced sanitary sewer overflows (SSOs) and treatment
facilities, including hydraulic overloading and disruptions of biological and other plant processes. As agencies examine
alternative approaches to controlling I/I problems, storage facilities are increasingly being planned, designed, and
constructed for the control of wet weather flows in sanitary sewer systems. While storage facilities have commonly been
constructed at wastewater treatment facilities (where they are referred to as equalization basins) to maximize the processing
of wastewater generated during wet weather while protecting the plant processes from hydraulic overloading or biological
disruption, the use of storage at upstream locations within the collection system has only recently begun to gain acceptance.
Collection system storage facilities are now being recognized as providing much of the benefit to treatment facilities realized
with equalization basins, with the additional benefit of controlling SSOs and basement flooding while minimizing or eliminating
the need to construct relief sewers.
Wet weather flow storage facilities may be constructed inline or offline. These facilities can be constructed inland and
upstream, on the shoreline, or in the receiving water. In addition to storage, other functions of these facilities may
include sedimentation (and associated toxics removal), dryweather flow equalization, flood protection, flow attenuation to
enhance receiving stream assimilation, and hazardous material spills capture. The development of new and improved concepts
for wet weather flow treatment and control is covered in NRMRL's Wet Weather Flow Research Plan. This plan predominantly
addresses the structural aspects of stormwater and wastewater storage facilities. Conventional facilities are usually
concrete or earthen basins or impoundments. During the last two years alone, hundreds of lives were lost, thousands of
square miles of ecosystems were destroyed or devastated, and $50 billion of property damage was caused by the failure of
these types of facilities due to excessive stormwater. Besides these conventional wet weather flow related facilities,
there are as many as 500,000 earthen-diked impoundments (pits, ponds and lagoons) in the United States containing potentially
hazardous wastes. This total includes small waste ponds at minor chemical manufacturing plants as well as mile-square tailing
lagoons at mines, smelters, and phosphoric acid plants. A common element between these similar systems is that a seemingly
secure earthen impoundment may suddenly fail with provocation as slight as a heavy rain. These failures have resulted in
the loss of life, caused the uncontrolled release of contaminants, polluted waterways, killed aquatic species, adversely
impacted drinking water systems, generated public and political concerns, and despoiled scenic areas.
Research Problem...
Earthen impoundments, levees and dams generally consist of an embankment (with an upstream and downstream slope) and a base
foundation. Such systems are constructed of a variety of soils; frequently, with the materials that were at hand. The resulting
structures may be susceptible to sudden failure. Additionally, seepage may also reduce slope strength and contribute to failure,
because it brings fluid to the downstream slope and thereby adds weight, increases the load, and decreases apparent cohesiveness
and effective normal force. Failure through the base must be considered, particularly when the soil beneath the dam is softer
than the slope-forming soil. In addition, unless adequate freeboard (embankment height above the maximum expected fluid level)
is provided, failure can occur through overtopping. Earthen impoundments are subject to overtopping and failure through the
collection of rainwater, run-off or other uncontrolled inflow. Overflows are generally the result of insufficient capacity
due either to insufficient freeboard or structural problems that reduce the effective capacity. No matter what the cause,
impoundment overflows of hazardous wastescan pose significant environmental and public health risks through the contamination
of soils, ground water, and surface water, as well as the potential to spread the contamination into areas not currently
impacted by the impoundment.
Because this problem involves thousands of earthen impoundments and dams, and tens of thousands of levee-miles (six thousand
in northern California alone) throughout the country, an inexpensive and simple technique for monitoring the stability of
earthen dams is needed. The high cost of conventional methods of monitoring dam stability usually rules them out as candidates
for evaluating failure potential of small waste dams. It seems unlikely that conventional methods of inspection, which
require expensive instrumentation and trained geo-technical engineers, can meet the need. Under this impetus, EPA ORD developed
a prototypical acoustic emission monitoring system -- based on the phenomenon that soils emit sounds under stress. Initial
research results demonstrated that the resulting acoustical signals, when properly amplified and quantified, can be valuable
guides in evaluating the stability of earthen impoundments. Recent advances in signal processing, computer
hardware and software, and telemetry now provide a cost-effective platform from which these initial research efforts can
be developed into an accurate, reliable and inexpensive system for monitoring the stability of earthen structures. Such
systems will provide real-time data regarding the structural condition of remote earthen structures. This is especially
critical during emergency situations since it would enable responsible officials to deploy limited resources to reinforce
the most critical areas and avert catastrophic failures.
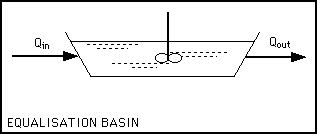
"Definition Sketch for Volumetric Feed Rate Equalization"...
Click here for the "Example for Equalization"...
Equalization in the Activated Sludge Process - An Example...
Activated Sludge - No Action Alternative...
For the Activated Sludge/No Action alternative (No Action alternative), the SBIWTP (South Bay International Wastewater
Treatment Plant) would have the same activated sludge secondary treatment as selected in the 1994 Final EIS. This
alternative assumes that Mexico will manage the wastewater flows to provide a constant flow of 25 mgd (1,095 L/s) to
the SBIWTP; thus, the constant flow through both primary and secondary treatment would be 25 mgd (1,095 L/s).
Mexico would be responsible for peak flows above 25 mgd (1,095 L/s). Construction and operation of these facilities
were approved in the 1994 Final EIS and ROD for the SBIWTP project. The proposed new activated sludge and related facilities
are sized to treat an average monthly organic loading of 370 milligrams per liter (mg/L) 5day biochemical oxygen demand
(BOD5), 350 mg/L total suspended solids (TSS), and an average flow of 25 mgd (1,095 L/s). BOD5
and TSS would be reduced to 19 mg/L each in the effluent from this alternative.
SBIWTP with Activated Sludge Secondary Treatment...
This alternative comprises activated sludge secondary treatment at the SBIWTP to accommodate an average flow of 25 mgd
(1,095 L/s) with options for treating peak flows. The first option involves the construction of a flow equalization
basin to accommodate peak flows up to 50 mgd (2,190 L/s). The second option under this alternative involves an increase
in the capacity of the secondary facility at the SBIWTP to treat peak flows up to 50 mgd (2,190 L/s).
Activated Sludge with Flow Equalization Basin...
This option would result in an average flow of 25 mgd (1,095 L/s) into the SBIWTP with a flow equalization basin
to accommodate peak flow storage and subsequent off-peak discharge to the secondary activated sludge facility. A flow
equalization basin capable of storing advanced-primary-treated peak flows greater than 25 mgd (1,095 L/s) would
be constructed for this alternative. A storage volume of 7 million gallons (MG) would be required. Accordingly, the
average flow through both the advanced primary and secondary portions of the plant would be 25 mgd (1,095 L/s).
Flow through the advanced primary portion of the plant is projected to follow the identified daily flow variations with a
low flow from 3.5 mgd (153 L/s) to a peak flow of 50 mgd (2,190 L/s). Before this variable flow enters
the secondary facility, it will be equalized by the basin to a steady rate of 25 mgd (1,095 L/s). The flow
equalization basin would be located within the existing footprint of the SBIWTP. Other than the flow equalization basin,
construction and operation of these facilities were addressed in the 1994 Final EIS and ROD. (A smaller flow equalization
basin sized at 5.5 mg, however, was considered as part of the 1997 Final Interim Operation SEIS.) These proposed new
activated sludge and related facilities are sized to treat a monthly average organic loading of 370 mg/L BOD5
and 350 mg/L TSS, and an average flow of 25 mgd (1,095 L/s). The equalization basin facilities are designed to
equalize flows to a constant 25 mgd (1,095 L/s). The activated sludge facilities are designed to provide an
effluent quality of 19 mg/L BOD5 and 19 mg/L TSS.
Activated Sludge with Expanded Capacity...
For this alternative, the secondary facility would be sized to treat peak flows up to 50 mgd (2,190 L/s). The
number of secondary clarifiers would be doubled from 8 to 16 to accommodate these peaks. Thus, an average flow of 25 mgd
(1,095 L/s) with peak flows up to 50 mgd (2,190 L/s) will be treated by both the advanced primary and secondary
facilities. The proposed new facilities would be located on the existing footprint of the SBIWTP and on a portion of the
Hofer site. Construction and operation of these facilities were addressed in the 1994 Final EIS and ROD. These proposed new
activated sludge and related facilities are sized to treat an average monthly organic loading of 370 mg/L BOD5,
350 mg/L TSS, and an average flow of 25 mgd (1,095 L/s). These facilities are designed to treat peak flows of
50 mgd (2,190 L/s). The activated sludge facilities would be designed to provide an effluent quality of 19 mg/L
BOD5 and 19 mg/L TSS.
SBIWTP with Ponds Secondary or Secondary-Equivalent Treatment...
This alternative includes two treatment pond options capable of treating a 25-mgd (1,095 L/s) average flow with peaks
up to 50 mgd (2,190 L/s). In this alternative, conventional primary treatment, as opposed to advanced primary
treatment, would be provided at the SBIWTP to optimize the pond processes. In conventional primary treatment, settling
would occur without chemicals to assist that process. The primary effluent would be the influent to the pond systems. The
wastewater would be treated in the pond systems to a secondary or secondary-equivalent level. One option under this alternative
is a Completely Mixed Aerated (CMA) system at the Hofer site. The second pond treatment option is the Advanced Integrated Pond
System (AIPS) at the Spooner's Mesa site.
Neutralization...
Click on the link for "Definition Sketch"...
"Definition Sketch for Neutralization"...
Chemical reaction, according to the Arrhenius theory of acids and bases, in which a water solution of acid is mixed with
a water solution of base to form a salt and water; this reaction is complete only if the resulting solution has neither
acidic nor basic properties. Such a solution is called a neutral solution. Complete neutralization can take place when
a strong acid, such as hydrochloric acid, HCl, is mixed with a strong base, such as sodium hydroxide, NaOH. Strong acids
and strong bases completely break up, or dissociate, into their constituent ions when they dissolve in water. In the case
of hydrochloric acid, hydrogen ions, H+, and chloride ions, Cl-, are formed. In the case of sodium hydroxide, sodium ions,
Na+, and hydroxide ions, OH-, are formed. The hydrogen and hydroxide ions readily unite to form water. If the number of
hydrogen ions in the hydrochloric acid solution is equal to the number of hydroxide ions in the sodium hydroxide solution,
complete neutralization occurs when the two solutions are mixed. The resulting solution contains sodium ions and chloride
ions that unite when the water evaporates to form sodium chloride, common table salt. In a neutralization reaction in which
either a weak acid or a weak base is used, only partial neutralization occurs. In a neutralization reaction in which both
a weak acid and a weak base are used, complete neutralization can occur if the acid and the base are equally weak. The heat
produced in the reaction between an acid and a base is called the heat of neutralization. When any strong acid is mixed
with any strong base, the heat of neutralization is always about 13,700 calories for each equivalent weight of acid and
base neutralized. See article on pH; titration.
Acids and Bases...
Two related classes of chemicals; the members of each class have a number of common properties when dissolved in a solvent,
usually water. 1. Properties : Acids in water solutions exhibit the following common properties: they taste sour; turn
litmus paper red; and react with certain metals, such as zinc, to yield hydrogen gas. Bases in water solutions exhibit
these common properties: they taste bitter; turn litmus paper blue; and feel slippery. When a water solution of acid is
mixed with a water solution of base, water and a salt are formed; this process, called neutralization, is complete only
if the resulting solution has neither acidic nor basic properties. 2. Classification : Acids and bases can be classified
as organic or inorganic. Some of the more common organic acids are: citric acid, carbonic acid, hydrogen cyanide, salicylic
acid, lactic acid, and tartaric acid. Some examples of organic bases are: pyridine and ethylamine. Some of the common
inorganic acids are: hydrogen sulfide, phosphoric acid, hydrogen chloride, and sulfuric acid. Some common inorganic bases
are: sodium hydroxide, sodium carbonate, sodium bicarbonate, calcium hydroxide, and calcium carbonate. 3. Acids, such as
hydrochloric acid, and bases, such as potassium hydroxide, that have a great tendency to dissociate in water are completely
ionized in solution; they are called strong acids or strong bases. Acids, such as acetic acid, and bases, such as ammonia,
that are reluctant to dissociate in water are only partially ionized in solution; they are called weak acids or weak bases.
Strong acids in solution produce a high concentration of hydrogen ions, and strong bases in solution produce a high
concentration of hydroxide ions and a correspondingly low concentration of hydrogen ions. The hydrogen ion concentration
is often expressed in terms of its negative logarithm, or pH (see separate article). Strong acids and strong bases make
very good electrolytes (see electrolysis), i.e., their solutions readily conduct electricity. Weak acids and weak bases
make poor electrolytes. 4. See buffer; catalyst; indicators, acid-base; titration. 5. Acid-Base Theories : There are three
theories that identify a singular characteristic which defines an acid and a base: the Arrhenius theory, for which the
Swedish chemist Svante Arrhenius was awarded the 1903 Nobel Prize in chemistry; the Brönsted-Lowry, or proton donor,
theory, advanced in 1923; and the Lewis, or electron-pair, theory, which was also presented in 1923. Each of the three
theories has its own advantages and disadvantages; each is useful under certain conditions. 6. The Arrhenius Theory :
When an acid or base dissolves in water, a certain percentage of the acid or base particles will break up, or dissociate
(see dissociation), into oppositely charged ions. The Arrhenius theory defines an acid as a compound that can dissociate
in water to yield hydrogen ions, H+, and a base as a compound that can dissociate in water to yield hydroxide
ions, OH- . For example, hydrochloric acid, HCl, dissociates in water to yield the required hydrogen ions,
H+, and also chloride ions, Cl- . The base sodium hydroxide, NaOH, dissociates in water to yield the
required hydroxide ions, OH-, and also sodium ions, Na+. 7. The Brönsted-Lowry Theory : Some
substances act as acids or bases when they are dissolved in solvents other than water, such as liquid ammonia. The
Brönsted-Lowry theory, named for the Danish chemist Johannes Brönsted and the British chemist Thomas Lowry, provides a
more general definition of acids and bases that can be used to deal both with solutions that contain no water and solutions
that contain water. It defines an acid as a proton donor and a base as a proton acceptor. In the Brönsted-Lowry theory,
water, H2O, can be considered an acid or a base since it can lose a proton to form a hydroxide ion,
OH-, or accept a proton to form a hydronium ion, H3O+ (see amphoterism). When an acid
loses a proton, the remaining species can be a proton acceptor and is called the conjugate base of the acid. Similarly when
a base accepts a proton, the resulting species can be a proton donor and is called the conjugate acid of that base. For
example, when a water molecule loses a proton to form a hydroxide ion, the hydroxide ion can be considered the conjugate
base of the acid, water. When a water molecule accepts a proton to form a hydronium ion, the hydronium ion can be
considered the conjugate acid of the base, water. 8. The Lewis Theory : Another theory that provides a very broad
definition of acids and bases has been put forth by the American chemist Gilbert Lewis. The Lewis theory defines an acid
as a compound that can accept a pair of electrons and a base as a compound that can donate a pair of electrons. Boron
trifluoride, BF3, can be considered a Lewis acid and ethyl alcohol can be considered a Lewis base.
Salt...
Chemical compound (other than water) formed by a chemical reaction between an acid and a base (see acids and bases). 1.
Characteristics and Classification of Salts The most familiar salt is sodium chloride, the principal component of common
table salt. Sodium chloride, NaCl, and water, H2O, are formed by neutralization of sodium hydroxide, NaOH, a
base, with hydrogen chloride, HCl, an acid: HCl + NaOH -> NaCl + H2O. Most salts are ionic compounds (see
chemical bond); they are made up of ions rather than molecules. The chemical formula for an ionic salt is an empirical
formula; it does not represent a molecule but shows the proportion of atoms of the elements that make up the salt. The
formula for sodium chloride, NaCl, indicates that equal numbers of sodium and chlorine atoms combine to form the salt. In
the reaction of sodium with chlorine, each sodium atom loses an electron, becoming positively charged, and each chlorine
atom gains an electron, becoming negatively charged (see oxidation and reduction); there are equal numbers of positively
charged sodium ions and negatively charged chloride ions in sodium chloride. The ions in a solid salt are usually arranged
in a definite crystalline structure, each positive ion being associated with a fixed number of negative ions, and vice
versa. 2. A salt that has neither hydrogen (H) nor hydroxyl (OH) in its formula, e.g., sodium chloride (NaCl), is called a
normal salt. A salt that has hydrogen in its formula, e.g., sodium bicarbonate (NaHCO3), is called an acid salt.
A salt that has hydroxyl in its formula, e.g., basic lead nitrate (Pb[OH]NO3), is called a basic salt. Since a
salt may react with a solvent to yield different ions than were present in the salt (see hydrolysis), a solution of a
normal salt may be acidic or basic; e.g., trisodium phosphate, Na3PO4, dissolves in and reacts with
water to form a basic solution. 3. In addition to being classified as normal, acid, or basic, salts are categorized as
simple salts, double salts, or complex salts. Simple salts, e.g., sodium chloride, contain only one kind of positive ion
(other than the hydrogen ion in acid salts). Double salts contain two different positive ions, e.g., the mineral dolomite,
or calcium magnesium carbonate, CaMg(CO3)2. Alums are a special kind of double salt. Complex salts,
e.g., potassium ferricyanide, K3Fe(CN)6, contain a complex ion that does not dissociate in solution.
A hydrate is a salt that includes water in its solid crystalline form; Glauber’s salt and Epsom salts are hydrates. 4. Salts
are often grouped according to the negative ion they contain, e.g., bicarbonate or carbonate, chlorate, chloride, cyanide,
fulminate, nitrate, phosphate, silicate, sulfate, or sulfide. 5. Preparation of Salts : Salts are also prepared by methods
other than neutralization. A metal can combine directly with a nonmetal to form a salt; e.g., sodium metal reacts with
chlorine gas to form sodium chloride. A metal may react with a dilute acid to form a salt and release hydrogen gas; e.g.,
zinc reacts with dilute sulfuric acid to form zinc sulfate and hydrogen. A metal oxide may react with an acid to form a
salt and water; e.g., calcium oxide reacts with carbonic acid to form calcium carbonate and water. A base can react with
a nonmetallic oxide to form a salt and water; e.g., sodium hydroxide reacts with carbon dioxide to form sodium carbonate
and water. Two salts may react with one another (in solution) to form two new salts; e.g., barium chloride and sodium
sulfate react in solution to form barium sulfate (as an insoluble precipitate) and sodium chloride (which remains in
solution). A salt may react with an acid to form a different salt and acid; e.g., sodium chloride and sulfuric acid react
when heated to form sodium sulfate and release hydrogen chloride gas (which in solution forms hydrochloric acid). A salt
undergoes dissociation when it dissolves in a polar solvent, e.g., water, the extent of dissociation depending both on the
salt and the solvent.
Equivalent Weight...
The equivalent weight of an element or radical is equal to its atomic weight or formula weight divided by the valence it
assumes in compounds. The unit of equivalent weight is the atomic mass unit; the amount of a substance in grams numerically
equal to the equivalent weight is called a gram equivalent. Hydrogen has atomic weight 1.008 and always assumes valence 1
in compounds, so its equivalent weight is 1.008. Oxygen has an atomic weight of 15.9994 and always assumes valence 2 in
compounds, so its equivalent weight is 7.9997. The sulfate radical (SO4) has formula weight 106.062 and always
has valence 2 in compounds, so its equivalent weight is 53.031. Some elements exhibit more than one valence in forming
compounds and thus have more than one equivalent weight. Iron (atomic weight 55.847) has an equivalent weight of 27.924 in
ferrous compounds (valence 2) and 18.616 in ferric compounds (valence 3). The weight proportion in which elements or
radicals combine to form compounds can be determined from their equivalent weights. For example, hydrogen can combine with
oxygen to form water; the weight proportion of oxygen to hydrogen in water is the same as the proportion of their equivalent
weights, 7.9997 to 1.008 or 7.946 to 1; there is 1 weight of hydrogen for every 7.946 weights of oxygen, or water is about
11.2% hydrogen (by weight). Iron forms two oxides: ferrous oxide (FeO), in which there are 27.924 weights of iron for each
7.9997 weights of oxygen, and ferric oxide (Fe2O3), in which there are 18.616 weights of iron for
every 7.9997 weights of oxygen.
Titration...
Gradual addition of an acidic solution to a basic solution or vice versa (see acids and bases); titrations are used to
determine the concentration of acids or bases in solution. For example, a given volume of a solution of unknown acidity
may be titrated with a base of known concentration until complete neutralization has occurred. This point is called the
equivalence point and is generally determined by observing a color change in an added indicator such as phenolphthalein.
From the volume and concentration of added base and the volume of acid solution, the unknown concentration of the solution
before titration can be determined. Titrations can also be used to determine the number of acidic or basic groups in an
unknown compound. A specific weight of the compound is titrated with a known concentration of acid or base until the
equivalence point has been reached. From the volume and concentration of added acid or base and the initial weight of the
compound, the equivalent weight, and thus the number of acidic or basic groups, can be computed. Instead of adding an
indicator to observe the equivalence point, one can construct a graph on which the pH (see separate article) at regular
intervals is plotted along one axis and the number of moles of added acid or base at these intervals along the other axis;
such a plot is called a titration curve and is usually sigmoid (S-shaped), with the inflection point, where the curve
changes direction, corresponding to the equivalence point. From the pH at the equivalence point, the dissociation constant
of the acidic or basic group can be determined (see chemical equilibrium). If a compound contains several different acidic
or basic groups, the titration curve will show several sigmoid-shaped curves like steps and the dissociation constant of
each group can be obtained from the pH at its corresponding equivalence point.
pH Neutralization For Pollution Control...
Objectives : 1. Acid and Base Recognition. 2. Neutralization of Acid and Base Chemicals for Pollution Control. 3.
Practical Application For Chemical Industries.
Materials needed : Vinegar (Acetic acid), Baking Soda, Distilled Water, Hydrogen peroxide, Detergent, All purpose
cleaner, Industrial effluent, Caustic lye (NaOH), pHydrion paper, Ammonia, Lime, Beakers, Test Tubes, Stirrers.
Strategy : Divide whole class into four groups. Each person from respective group takes vinegar solution in beaker
about 10 mL. Each group should also collect pHydrion paper. Then each group has to dip pHydrion paper into vinegar solution
and observe the color. From the color of the pHydrion paper, each group will decide pH numerical value by referring to the
color chart. Each group should record the pH value in record data sheet. From pH value, each group will be able to decide
whether vinegar solution is acidic or alkaline.
Second chemical solution to be given to each group is washing soda. Repeat the same procedure.
Third chemical solution to be given to each group is hydrogen peroxide. Repeat the same procedure.
Then the teacher demonstrates dilute hydrochloric acid to show its pH value, that is acidic.
Next, pH of mild detergent is to be tested, that is almost neutral.
Other solutions we tested were all purpose cleaner, ammonia, caustic lye, and industrial effluent.
Thereafter ask each group to make a chart. Let them prepare three columns, one for acid, one for base, and one for
neutral. Discussed results with students. Have students continue filling results in data chart.
After testing industrial effluent class discussed consequences of its disposal to sewer system.
Take 20 mL of hydrochloric acid in beaker. Add same amount of lime (CaCO3) into beaker. Stir it and measure
its pH value. If mixed solution is not achieving pH value as 7 then keep on adding lime solution until it achieves pH
value as 7. At this point, titration is said to be completed. Explain to students that quantity of lime material required
for neutralization depends on concentration of acid.
After this, explain to the students that is why lime is commonly used for neutralizing industrial effluent. The reasons
explained were that lime is a strong base, economical, and most to use.
Second neutralization process shown to students was caustic lye, and strong hydrochloric acid.
Application : Use overhead projector to show transparency of standard model of small scale industry pollution
treatment plant, explain its operation, and design.
Conclusion : During enrichment sessions, we had discussed the importance of neutralization process. Students
explained that if industrial effluent is not neutralized then effluent will corrode the sewer pipelines and it will
penetrate inside the soil. Seepage of effluent remains as ground water so when a tube well is used, the water will come
out polluted. This polluted water is toxic and harmful for domestic purpose.
Health Effects of Acids and Bases...
Acids and bases are examples of corrosive poisons which react locally on tissue cells. Chemicals that are very basic or
very acidic are reactive. These chemicals can cause severe burns. Automobile battery acid is an acidic chemical that is
reactive. Automobile batteries contain a stronger form of some of the same acid that is in acid rain. Household drain
cleaners often contain lye, a very alkaline chemical that is reactive. Acids and bases are capable of causing severe
"burns" similar to burns produced by heat. These materials act by first dehydrating cellular structures. Then proteins
structures are destroyed by the action of acid or base which catalyzes the splitting of peptide bonds. Smaller and smaller
fragments result, leading to the ultimate disintegration of the tissue. The eyes and lungs are particularly sensitive to
corrosive poisons. The cornea of the eyes is damaged by acid or base burns. Pulmonary edema (filling with water) occurs
when highly concentrated corrosive pollutants (acute poisoning) reach the lungs. The lining of the nose, sinuses and lungs
become irritated and water logged (by dehydration of cells). This occurs in an attempt to dilute the toxic agent. This
waterlogged condition prevents the normal exchange of oxygen and carbon dioxide. The victim may die of immediate
suffocation, a secondary attack of bacteria leading to pneumonia, or suffer permanent lung damage. Long term subchronic
acid-base dosage effects are less well understood. Air pollutants such as sulfur oxides, nitrogen oxides, chlorine, and
ammonia all have corrosive effects on the respiratory tract. The solubility of the gases in water determines their fate.
The most soluble gases, such as ammonia and sulfur oxides, are promptly adsorbed to the moist surfaces of the upper airways
causing nasal and throat irritation. Less soluble gases, such as nitrogen oxides and chlorine, produce their effects in the
depths of the lungs causing pulmonary edema, pneumonia, and emphysema (loss of elasticity and surface area).