Chemical Treatment - 02...
Chapter 2 - Precipitation General Discussion and Theory...
2-1. Introduction...
All precipitation processes operate under the same fundamental chemical principles. Precipitation is a physicalchemical
process, in which soluble metals and inorganics are converted to relatively insoluble metal and inorganic salts
(precipitates) by the addition of a precipitating agent. Most often, an alkaline reagent is used to raise the solution pH
to lower the solubility of the metallic constituent, and, thus, bring about precipitation.
( a ) For example, using caustic soda as the precipitating agent to lower the amount of soluble nickel by forming nickel hydroxide precipitate ("s" denotes solid precipitate) creates the following reaction :

Precipitates, which are small or colloidal, are then coagulated, flocculated, settled, clarified, or filtered out of
solution, leaving a lower concentration of metals and inorganics in the effluent. Figure 2-1 is a typical schematic of a
metals P/C/F system.
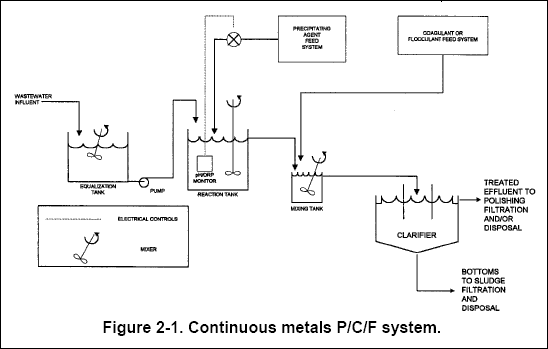
( b ) Chemical precipitation depends on several variables, including :
Maintenance of a proper pH range throughout the precipitation reaction and subsequent settling time.
Addition of a sufficient excess of treatment ions (precipitating agent) to drive the precipitation reaction to completion.
Effective removal of precipitated solids.
To effectively precipitate metals, control of pH is essential (especially true for hydroxide precipitation, see Chapter 3),
as illustrated by the solubility curves for selected metal-hydroxides and metal-sulfides shown in Figure 2-2.
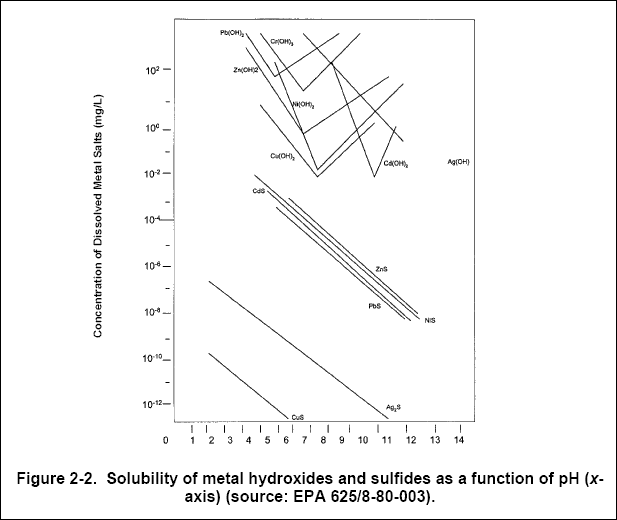
( c ) Hydroxide precipitation effectively removes cadmium, chromium(+3), copper, iron, manganese, nickel, lead, and zinc
(EPA, 1987). Sulfide precipitation effectively removes cadmium, chromium (+6), cobalt, copper, iron, mercury, manganese,
nickel, silver, tin, and zinc. Carbonate precipitation effectively removes nickel, cadmium, lead and zinc.
( d ) Theoretical solubilities of selected metal-hydroxides, sulfides, and carbonates are listed in Table 2-1. Solubility
is defined as the number of moles (or milligrams) of a solid (precipitate) that will dissolve in a liter of solution. This
is an important concept to the designer, as it dictates the theoretical minimum concentration of soluble metals that will
be present in the supernatant (clear effluent water) after the precipitation and clarification.
2-2. Theory and Discussion...
Precipitation alters the ionic equilibrium of a metallic compound to produce a relatively insoluble precipitate.
In other words, it means inducing supersaturated conditions (i.e., the solubility-product constant, typically denoted
as KSP, is exceeded). For the solid precipitate CaAb (s), the following general
solubility expression can be written :
CaAb (s) = ( a ) ( C ) + ( b ) ( A )
Where ;
KSP = [ C ]a [ A ]b
and [ A ] and [ C ] are in moles per liter. Note that solid precipitates do not enter into the solubility-product
constant calculation. Ksp represents the maximum value the product that the ion concentrations can have at equilibrium
conditions for a given temperature. Therefore, for precipitation to take place, supersaturated conditions (non-equilibrium,
by definition) must be present.
( a ) Given the following example equation, where Ni( OH )2 is a solid precipitate :
Ni( OH )2 (s) = Ni + 2 + 2 OH -
Where ;
KSP = [ Ni + 2 ] [ OH - ] 2 and KSP = 1.6 Χ 10 - 16,
at 25 O C (Benefield et al., 1982). There exist two corollary statements, which relate to the solubility-product
constant principle, that explain the phenomena of precipitation and solution of precipitates. These statements are as
follows : ( 1 ) Unsaturated Solution. In an unsaturated solution, the product of the molar concentrations of the ions is
less than the solubility-product constant, or [ Ni + 2 ] [ OH - ] 2 < KSP.
In this case, if undissolved Ni( OH )2 is present, it will dissolve to the extent that [ Ni + 2 ]
[ OH - ] 2 = KSP. ( 2 ) Supersaturated Solution. In a supersaturated solution, the
product of the molar concentrations of the ions is greater than the solubility-product constant, or [ Ni + 2 ]
[ OH - ] 2 > KSP. In this case, if internal forces allow formation of crystal nuclei,
then precipitation will occur until the ionic concentrations are reduced equal to those of a saturated solution.
( b ) The designer should be aware that the relative solubilities of compounds cannot be predicted by a simple comparison
of the solubility-product constant values because of the squares and cubes that enter into the calculation. See Table 2-2,
which gives solubility-product constant values and solubility values for examples of different types of precipitates. For
example, note that the solubility-product constant, KSP, for Cr ( OH ) 3 is greater than
Ca 3 ( PO 4 ) 2 ; however, Cr ( OH ) 3 is less (more than 10 times less)
soluble than Ca 3 ( PO 4 ) 2 . It is important to closely examine the units used in the
literature, as solubility is expressed in both moles/L and mg/L. A mole of a substance is its gram molecular weight (e.g.,
1 mole of zinc is 65.4 g).
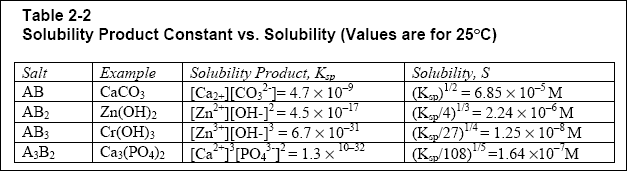
( c ) Removal efficiencies (or solubilities) observed in actual practice will often differ (both higher and lower)
considerably from theoretical solubilities. In most cases, actual solubilities will be greater than theoretical solubilities
because of incomplete reactions, poor separation of colloidal precipitates, and the formation of soluble metal-complexes
(metal-chelates) not considered in the equilibrium model. However, actual solubilities may be lower than theoretical
solubilities because of coprecipitation (Benefield et al., 1982).
( d ) Owing to the difficulty of theoretically predicting actual solubilities, it is essential that jar testing be conducted
before the P/C/F system is designed to best simulate in-field conditions. Jar testing is discussed further in Chapter 10. A
summary of factors that influence the solubility of metal ions and precipitates is given below.
( 1 ) Complex Formation : Solubility relationships are generally much more complicated than what has been discussed
earlier. Complex formation in wastewaters or natural waters must be considered to make realistic solubility calculations.
Reactions of the cations or anions with water to form hydroxide complexes or protonated anion species are common. In
addition, the cations or anions may form complexes with other materials in solution, thus reducing their effective
concentration. Soluble molecules or ions, which can act to form complexes with metals, are called ligands. Common
ligands include OH , CO 3 - 2 , NH 3 , F , CN ,
S 2 O 3 - 2 , as well as numerous other inorganic and organic species. In complex
formation equilibria equations, the formation constant is also known as the instability constant (often denoted as
K I in the literature). Waste streams containing complexing/chelating agents are often untreatable with
established technologies (see Chapter 11). The following references discuss complex formation : Benefield et al. (1982),
and Anderson (1994).
( 2 ) Chelating Agents : The solubility of metal ions is also increased by the presence of chelating agents. A
chelating agent forms multiple bonds with the metal ion. These bonds essentially form a ring in which the metal ion is held
so that it is not free to form an insoluble salt. The "pinchers" of the chelating molecule consist of ligand atoms. Common
chelating agents are ethylenediamine tetraacetic acid (EDTA, see Figure 2-3, where cobalt is the metal ion), citrate, and
tartrate (see Chapter 11).
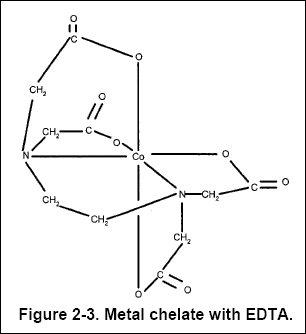
( 3 ) Temperature : Solubility depends on temperature; solubilities of inorganic and metal precipitates
generally increase with increasing solution temperatures. The designer should be aware that KSP and
KI values are valid for only a single temperature. References typically show KSP and
KI values at 25 O C. Ground water temperatures depend on geographical location and typically
range from 4 to 10 O C (40 to 50 O F), in the northern U.S., to 10 to 25 O C
(50 to 75 O F), in the southern U.S., in wells 1020 m deep (Tchobanoglous and Schroeder, 1985).
( 4 ) Coprecipitation : The actual solubilities of metal precipitates are lower than the theoretical solubilities
if coprecipitation occurs. When the presence and precipitation of other metals in solution aid in the removal of target
metals through surface adsorption, it is called coprecipitation. An example of this is improved cadmium removal by
adsorption onto calcium carbonate precipitates (Anderson, 1994). Coprecipitation is discussed further in the paragraph
6-3.
( 5 ) Oxidation / Reduction : Certain metals may require oxidation (e.g., Fe + 2 to Fe + 3 )
or chemical reduction (e.g., Cr + 6 to Cr + 3 ) to change the valence state so that a particular
precipitation method can be effective. Oxidation and reduction methods are further discussed in Chapter 11.
( e ) EPA lists the following advantages and limitations of the precipitation and coprecipitation processes :
( 1 ) Advantages :
Processes are reliable and well proven.
Processes are relatively simple.
( 2 ) Limitations :
Reagent addition must be carefully controlled to preclude unacceptable concentrations in the effluent.
Efficacy of the system requires that solids be adequately separated (e.g., clarification, flocculation, or filtration).
Process may generate hazardous sludge, requiring proper disposal.
Process can be costly, depending on the reagents used, and the required system controls, sludge disposal methods, and
operator time.
Process is not stable for large concentration variations in the influent.
Start-up and shut down times are longer than those for packed-bed and membrane processes.
( f ) Several precipitation methods are available for removing heavy metals. For industrial applications, at least seven
technologies have been demonstrated at full-scale, including the following :
Hydroxide precipitation.
Sulfide precipitation.
Carbonate precipitation.
Xanthate precipitation.
Combined precipitation.
Sodium borohydride (SBH) treatment.
Dithiocarbamate precipitation.
In addition, there are many other chemicals that have not been demonstrated at full-scale, such as polysaccharides, which
are believed to be effective in the removal of metals from wastewaters (EPA, 1989).
( g ) The following five precipitation processes are addressed within this Manual :
Hydroxide.
Sulfide.
Carbonate.
Xanthate.
Combined.