Design Examples...
1. Package Plant Extended Aeration...
Design an extended aeration package plant (prefabricated or pre-engineered) to treat a municipal wastewater flow of 125,000
L/d (33,000 gal/d). Solids retention typically ranges from 20 to 30 days; MLSS varies between 3,000 and 6,000 mg/L. The
Food to Microorganism ratio (F/M) typically varies between 0.05 to 0.30. Influent BOD and TSS will generally be about 250
mg/L. The dissolved oxygen (DO) concentration is in the 1.5 to 2.5 mg/L range and preferably will never be below 2.0 mg/L.
Coarse bubble aerators will be used. Detention time in the aeration tank will be one day.
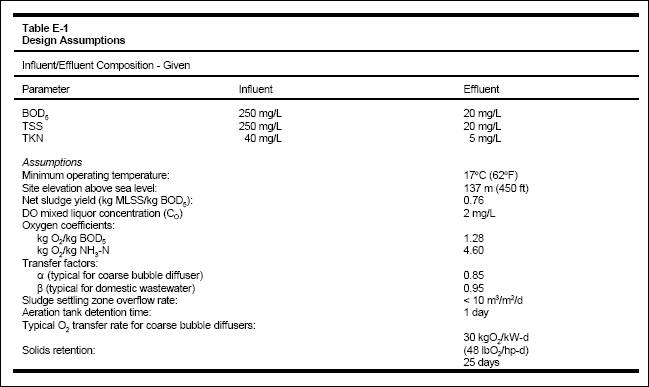
( a ) Sludge production.
Calculate the sludge production rate based on the desired BOD removal. Calculate the system total solids mass based on the
sludge production rate and the assumed solids retention time, as shown in Table E-2.
( b ) Aeration power.
Calculate the blower capacity based on the sludge production rate, desired TKN removal/synthesis, and the site specific
conditions, as shown in Table E-2.
( c ) Unit dimensions.
Estimate the required unit process dimemsions including the chlorine contact tank based on one-day hydraulic detention time
and using two sludge settling hoppers, as shown in Table E-2.
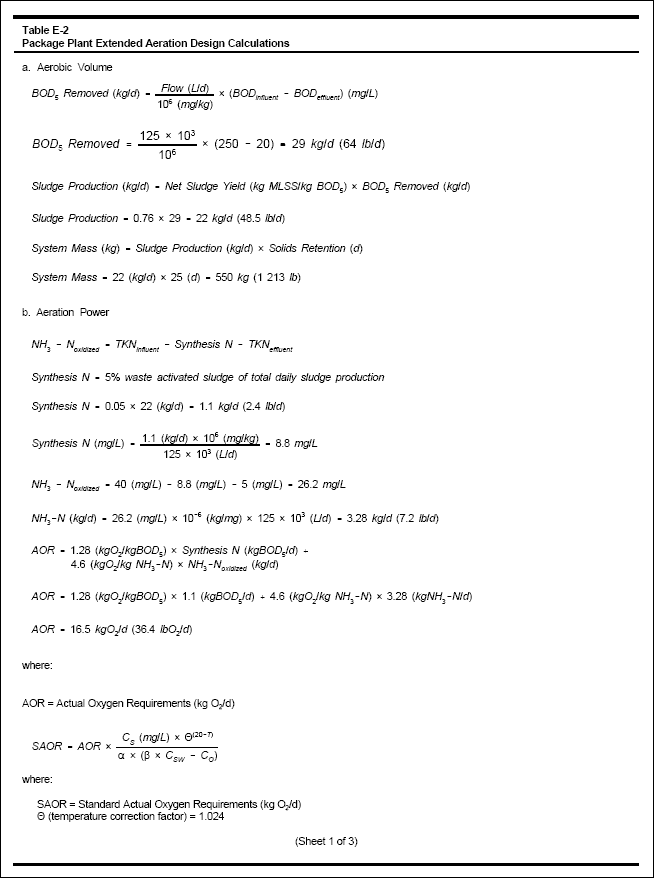
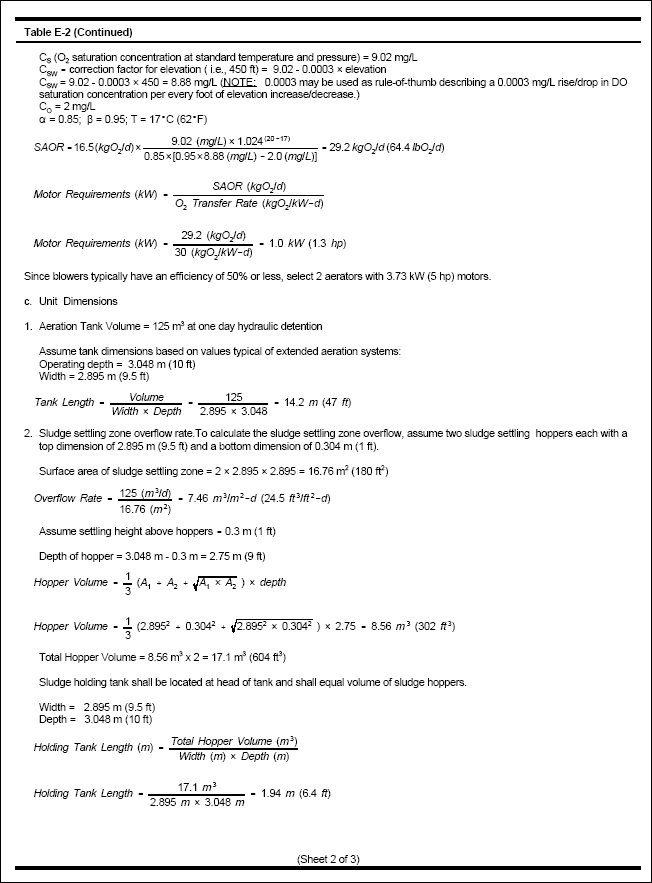
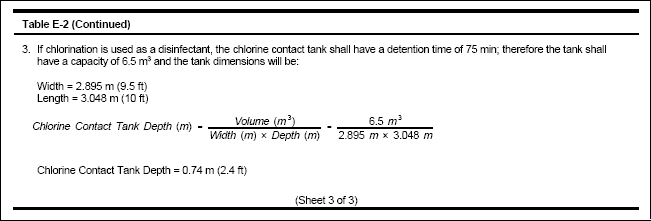
( d ) Equipment specifications.
Figure E-1 presents a plan view and a side view of the pre-engineered package plant extended aeration with the following
specifications :
(1) The unit package plant will require no pre-treatment other than wastewater pumping from an influent manhole lift
station.
(2) The influent pipe shall have a minimum of a 150 mm (6 in.) diameter from the influent manhole and will discharge
directly to a combination comminutor/bar screen located ahead of (and on top of) the aeration tank.
(3) Two 3.73 kW (5 hp) blower assemblies shall provide air at 31 kPa (4.5 psi) to ensure a 2.0 mg/L DO level in the
aeration tank at all times.
(4) A minimum of 44 diffusers will be required to distribute aeration at the aeration tank floor level. At least six (6)
diffusers will be provided in the sludge holding tank and one (1) in the chlorine contact tank.
(5) A totalizing flow meter will be provided to record the daily flow patterns and total.
(6) A minimum of eight spray nozzles will be required on the top of the aeration tank on the side opposite to the aeration
diffuser drops.
(7) Each sludge hopper will be equipped with an air lift pump with openings 150 mm (6 in.) above the hopper bottoms.
(8) The air lift pumps will discharge to a combination 75 mm (3 in.) sludge return and sludge waste line to the head of the
tank.
(9) Blower units shall be controlled by a blower panel located above the aeration tank.
(10) Scum skimmers will be provided at a scum baffle ahead of the tank discharge (by V-notch weir) to the chlorine contact
tank.
(11) Should ultraviolet disinfection be chosen in lieu of chlorination of tank effluent, an in-pipe rather than open channel
effluent flow may be specified.
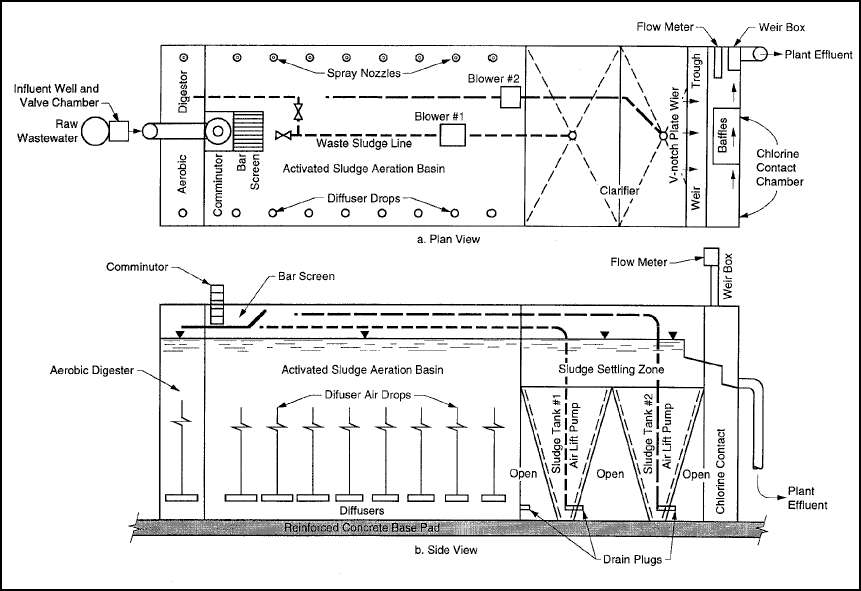
Figure E-1. Pre-engineered package plant extended aeration.
2. Oxidation Ditch (Continuous-Loop Reactor) Carrousel—Wraparound...
Design a carrousel (circular or wraparound) oxidation ditch to treat municipal wastewater at an average influent flow rate
of 378,500 L/d (100,000 gal/d). The new system will use mechanical aerators and have the design parameters shown in Table
E-3.
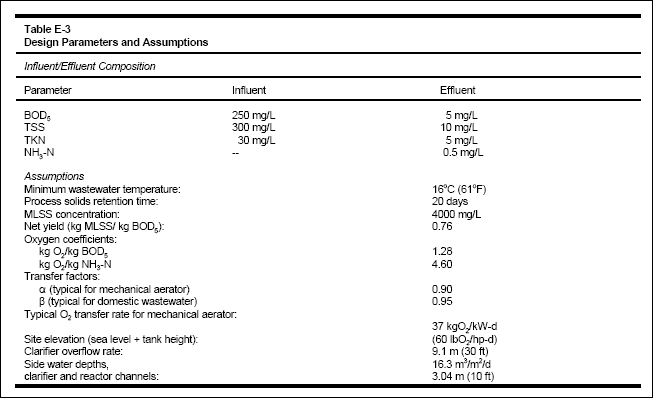
( a ) Carrousel volume.
Calculate the sludge production rate based on the desired BOD removal. Calculate the system total solids mass based on the
sludge production rate and the assumed solids retention time. Calculate the carrousel volume from the calculated system
total solids mass and the assumed MLSS concentration, as shown in Table E-4.
( b ) Aeration power.
Calculate the blower capacity based on the desired TKN removal/synthesis and the site specific conditions, as shown in
Table E-4.
( c ) Clarifier diameter.
Estimate the required wraparound clarifier diameter based on the assumed clarfier overflow rate and the side water depths,
as shown in Table E-4.
( d ) Carrousel specifications.
The carrousel shown in Figure E-2 has the following specifications :
- Clarifier diameter: 6.4 m (21 ft).
- Inner channel: 4 m (13 ft).
- Outer channel: 4 m (13 ft).
- Entire tank diameter: 14 m (46 ft).
- Walls and miscellaneous equipment thickness: 2 m (6.5 ft).
- Constructed carousel diameter: 14 m + 2 m = 16 m (52.5 ft).
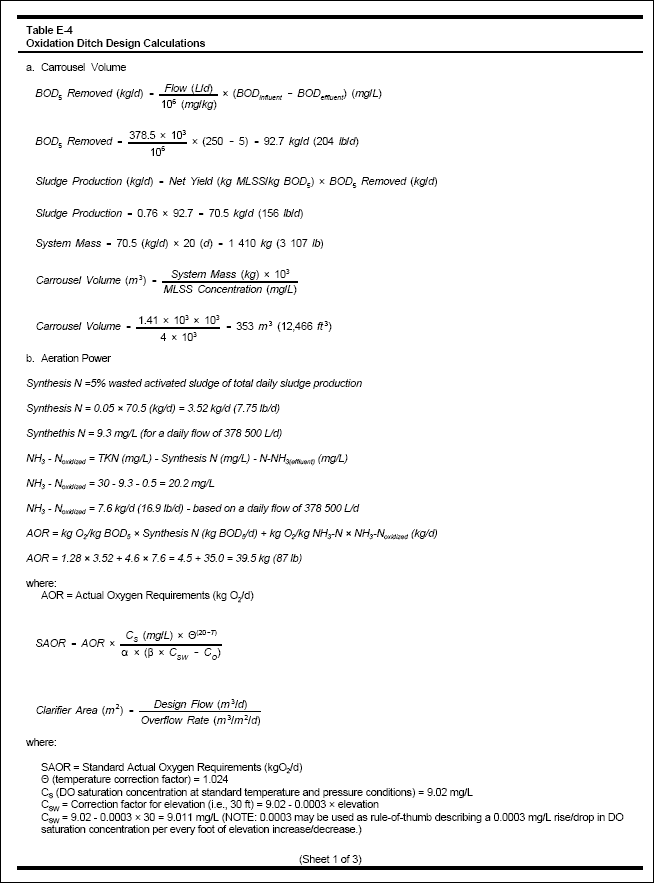
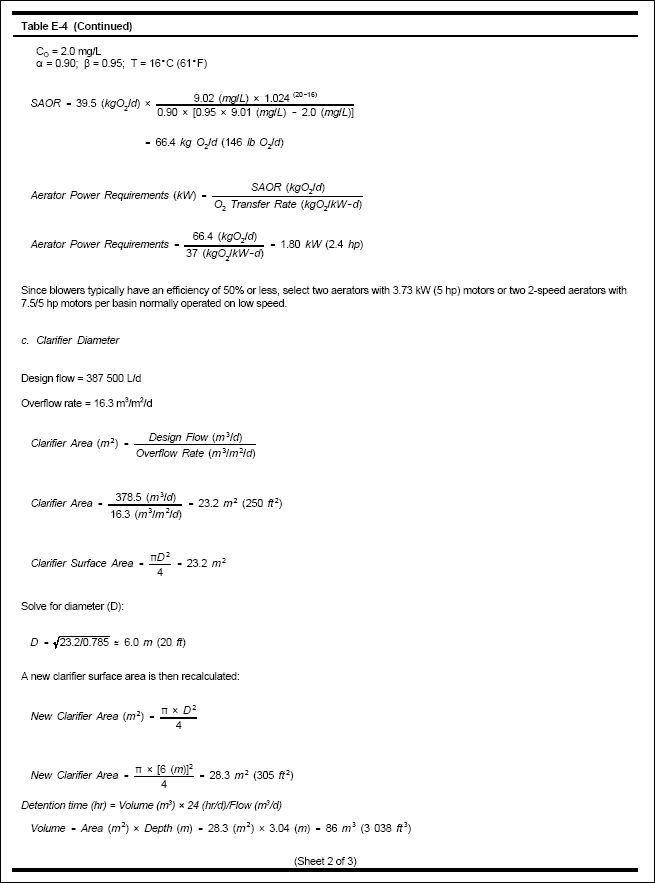
3. Stabilization Pond...
Design a facultative stabilization pond with primary treatment (clarifier and anaerobic digester of Imhoff tank design)
to be followed by secondary clarification to treat a domestic wastewater flow of 378,500 L/d (100,000 gal/d). Influent
BOD 5 will be 250 mg/L. Assume a primary clarifier removes 33 percent of the influent BOD 5
(BOD 5 = 0.68 BOD U ), and influent wastewater [SO 4 - 2 ] is <= 500 mg/L.
Four rectangular ponds in parallel are to be constructed. The controlling winter temperature of each pond will be 4.5
O C (40 O F). Length to width ratio of each pond will be 3:1, as is typical for such facilities.
Find, as shown in Table E-5 :
Total area of ponds.
Applied BOD loading.
Dimensions of the ponds.
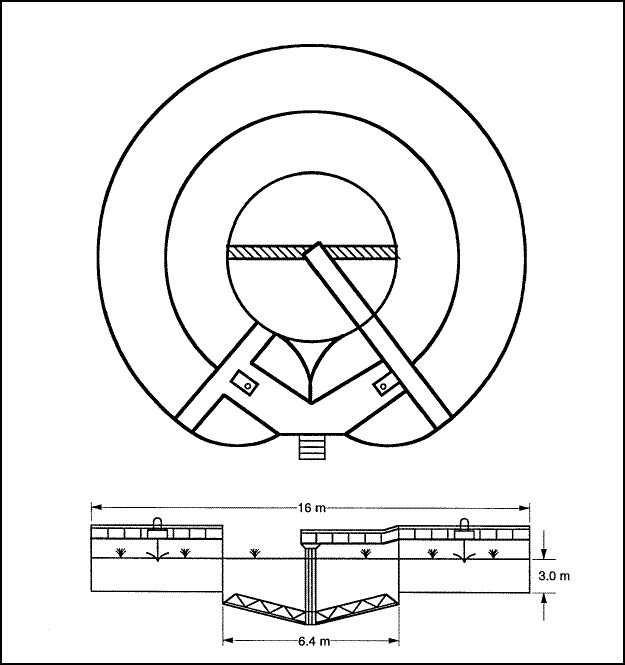
Figure E-2. Oxidation ditch carrousel wraparound (closed-loop reactor).
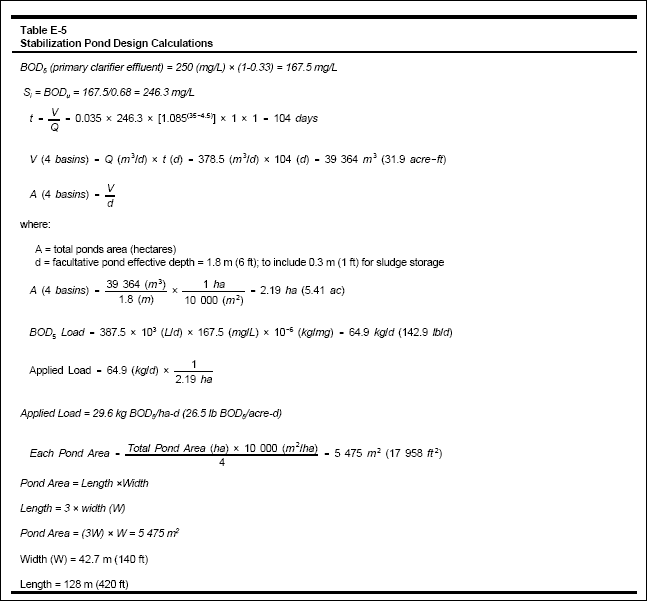
Use the Gloyna equation (“Facultative Waste Stabilization Pond Design in Ponds as a Wastewater Treatment Alternative,”
by E. F. Gloyna, J. F. Malina, Jr. and E. M. Davis) :
4. Zero Discharge or Water Recycle/Reuse For Toilet Flush Water in Rest Areas (Closed-Loop Reuse)...
( a ) Background.
(1) A combination of an extended aeration-activated sludge wastewater treatment system followed by
mixed-media pressure filtration has been successful in treating liquid waste from a comfort facility with
eight water closets and two urinals, plus lavatories. The design wastewater flow is 37,800 L/d (10,000
gal/d).
(2) The closed-loop reuse principle is generally applicable where liquid discharges from a recreational
area are not permitted or desired. After the system is initially filled and operational, a fraction of the
treated wastewater (about 6 percent) is fed to the terminal holding pond or lagoon to evaporate to account
for the makeup water used for lavoratories and drinking fountains. The makeup water is estimated to
represent about 6 percent of total water use. The sludge from the waste solids holding basin is periodically
removed by tank truck. The design parameters for the original extended aeration treatment system are
presented in Table E-6. Figure E-3 presents a schematic flow diagram of the wastewater recycle-reuse
system.
(3) It is to be expected that 90 to 95 percent of water used in a comfort station facility is for the water
closets or toilet flushing functions. Generally, 10 to 20 cycles are required for the system to reach equilibrium
with an input of 5 to 10 percent of potable water for the lavatories or drinking fountains. The
wastewater from lavatories and drinking fountains is considered “new” water and is a factor in the control
of the amount of wastewater that must be fed to the final holding pond to evaporate.
(4) Operating records reveal no objectionable odors from the water closets or lavatories, no
objectionable colors from blue (or other food dyes) introduced to give a sanitized look to the flushing
waters, no foaming in the sanitary facilities, and no building of total suspended solids. The 90 to 95 percent
of reused water in the water closets and urinals has an acceptable quality following the extended
aeration process and multimedia filtration.
(5) Use surveys to indicate that toilet flush water use is about 12.7 L (3.4 gal) per flush and 15.0 L
(4.0 gal) per toilet user. Potable water use (lavatories and drinking fountains) is approximately 0.8 L
(0.2 gal) per toilet user. Average resident time in the toilet facility is expected to be 3 min.
( b ) Recycled wastewater.
(1) The desired treatment characteristics of the recycled wastewater are shown in Table E-7.
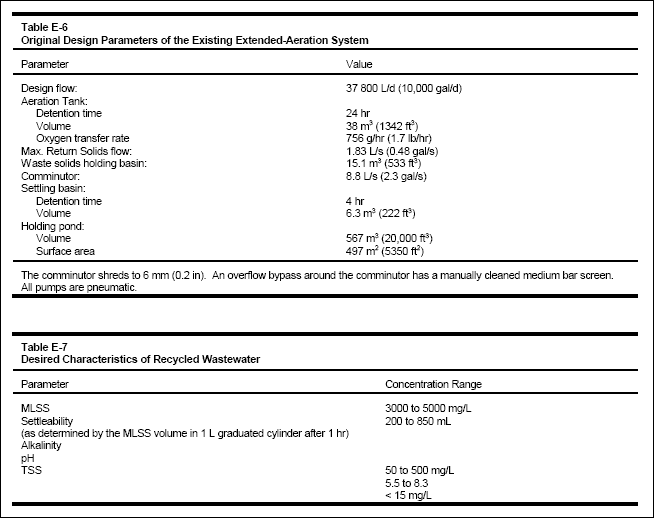
(2) To achieve the best operation, the recycled wastewater must be chemically stable and the total suspended solids and
total volatile solids must remain relatively constant. The most desirable range for MLSS would probably be 3,500 to 4,000
mg/L with an accompanying settleability of 400 to 600 mL.
( c ) Unit processes for closed-loop reuse.
(1) The unit processes shown in Table E-8 have been added for the closed-loop reuse to meet the desired characteristics
identified in Table E-7.
(2) The multimedia rapid filtration pressurized vessel has a design filtration rate of 80 to 160 L/min/m 2
and a backwash design flow rate of 285 to 610 L/min/m 2 . The filter appears to operate best at a filtration
rate of 94 L/min/m 2 (2.3 gal/min/ft 2 ) and at a backwash cleaning rate of 345
L/min/m 2 (8.5 gal/min/ft 2 ). Total suspended solids in the recycled wastewater must be less
than 15 mg/L for reuse in the toilet facility.
5. Sequencing Batch Reactor (SBR)...
( a ) General.
(1) The design of a sequencing batch reactor (SBR) involves the same factors commonly used for the flow-through activated
sludge system. The aspects of a municipally treated waste which require dentrification as well as nitrification plus
biological phosphorous removal need additional design considerations. Pretreatment of the wastewater before influent in
the SBR reactor system is also required.
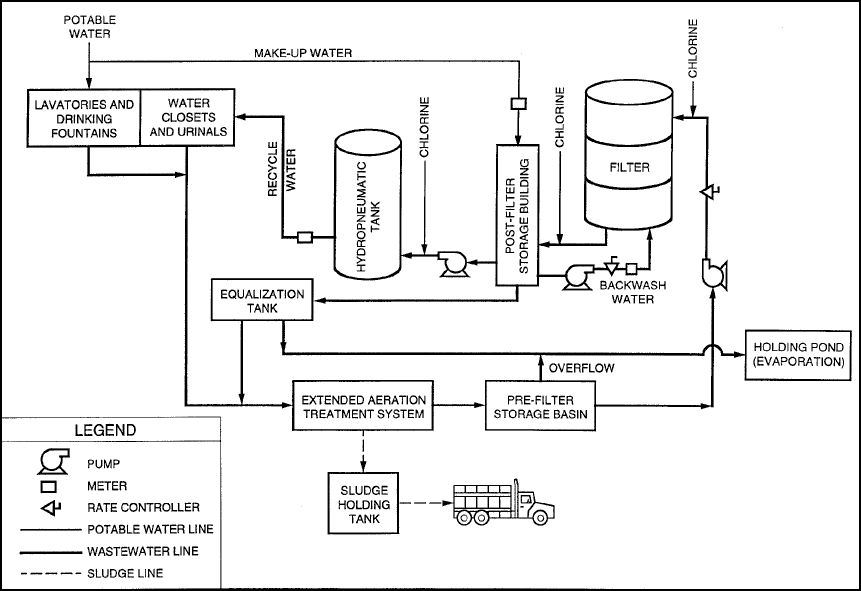
Figure E-3. Flow diagram for wastewater recycle-reuse system.
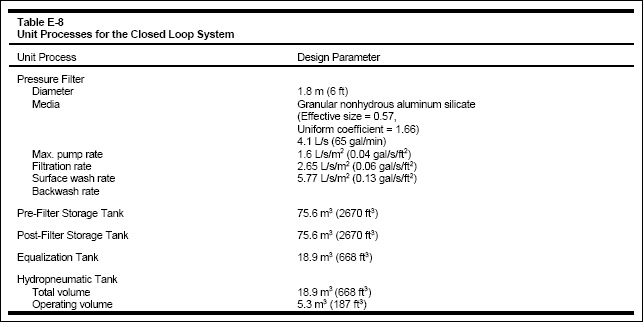
(2) The following example should be considered an outline to identify reactor volume elements, a
diffused aeration system, the basis for signing effluent decanter units, and waste sludge systems for a
system receiving 378,500 L/d (100,000 gal/d) of wastewater.
(3) Food-to-mass (F/M) ratio typically ranges from 0.05 to 0.30 with domestic waste F/M ratios
typically ranging from 0.10 to 0.15. At the end of the decant phase, the MLSS concentration may vary
between 2,000 and 5,000 mg/L. A typical value for a municipal waste would be 3500 mg/L. The MLSS
concentration changes continuously throughout an SBR operating cycle from a maximum at the beginning
of a fill phase to a minimum at the end of the react phase.
( b ) Reactor volume.
Calculate the reactor volume based on the desired BOD removal, the F/M ratio,
and the MLSS. The F/M ratio and the MLSS at the low water level determine the reactor volume at the
low water level, as shown in Table E-10.
( c ) Decant volume.
Calculate the decant volume as the difference between the reactor volume and
the low water volume, as shown in Table E-10. Each operating cycle is normally composed of mixed fill,
react fill, settle, decant, sludge waste, and idle. The number of cycles dictates the number of decants per
day or the volume of liquid to be decanted for each cycle. The volume per decant per cycle must be
selected based on the maximum sustained daily flow.
( d ) Detention time.
Calculate the maximum detention time based on the reactor volume. Calculate
the minimum detention time based on the decant volume, as shown in Table E-10.
( e ) SBR dimensions.
Estimate the required unit process dimensions, as shown in Table E-10. The
basin length can be estimated based on a recommended minimum depth. The minimum depth after decant
is determined as the depth of a clarifier in a flow-through system, i.e., quiescent settling and a large settling
area. A minimum depth of 2.75 m (9 ft) is typically recommended by designers.
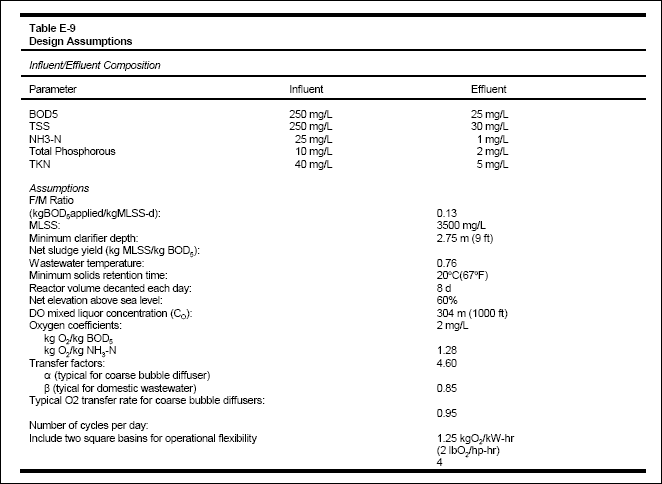
( f ) Aeration power.
Calculate the blower capacity based on the sludge production rate and the total
oxygen demand, as shown in Table E-10. In sizing the aeration equipment, it must be noted that the
equipment operates only a portion of the SBR operating cycle (part of the fill plus react phases).
Therefore, the total daily oxygen requirements must be met in a shorter time period than in a conventional
activated sludge flow-through system. The total daily oxygen requirements are estimated by adding the
carbonaceous oxygen demand (oxygen required for BOD oxidation) to the nitrogeneous oxygen demand
(oxygen required for TKN oxidation).
( g ) Sludge and decanter flows.
Calculate the sludge and decanter flow rates at design conditions, as shown in Table E-10.
( h ) Equipment specifications.
Figure E-4 presents a plan view of the SBR system with the following specifications :
(1) At least two basins are provided in an SBR design to provide operational flexibility and improved
effluent quality. SBR unit dimensions :
- Maximum volume = 470 m 3 (16,598 ft 3 ).
- High water level = 5.80 m (19 ft).
- Maximum decant height = 3.05 m (10 ft).
- Low water level = 2.75 m (9 ft).
- Hydraulic detention time at low water level = 17.9 hrs.
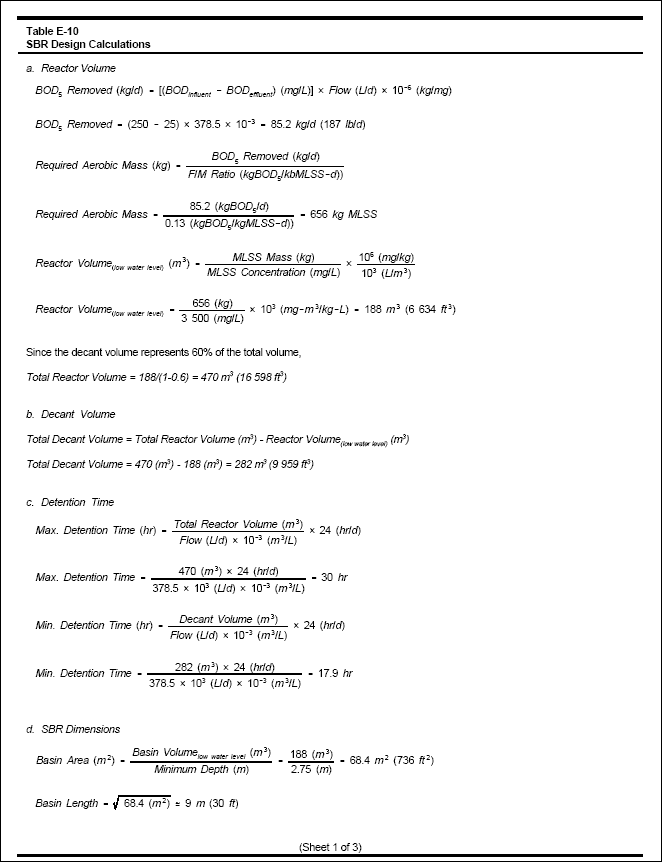
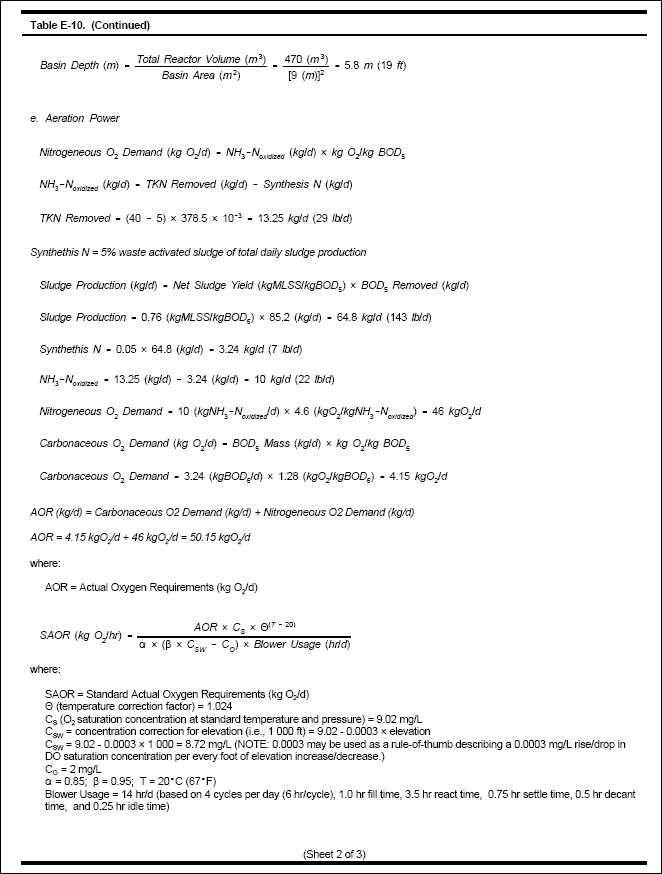
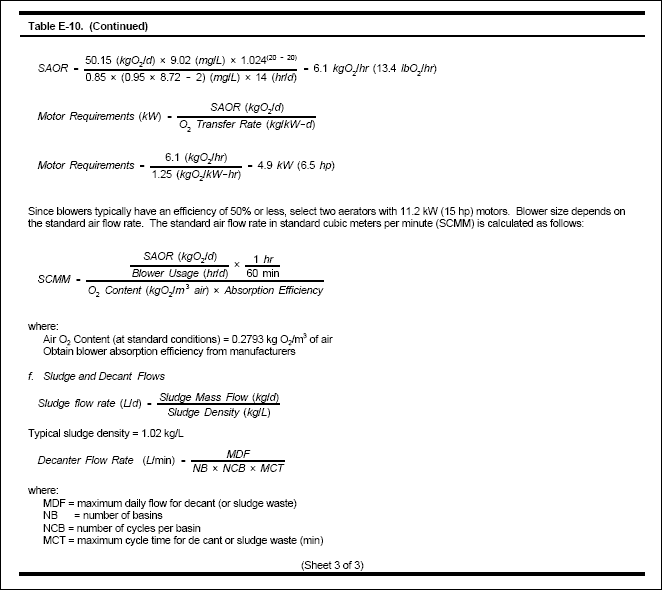
(2) Blowers: Rotary positive displacement.
(3) Diffusers: 4-10 tube coarse bubble retriever diffuser assembly (2 per basin).
(4) Mixers: 2 at 3.73 kW (5 hp).
(5) Sludge pumps: 2 at 1.49 kW (2 hp).
(6) Decanter sizing: Cycles per day = 4.
(7) Volume per decant = 70.5 m 3 (2,490 ft 3 ).
(8) Decant time = 30 min.
(9) Decant flow rate = 2.35 m 3 /min (621 gal/min).
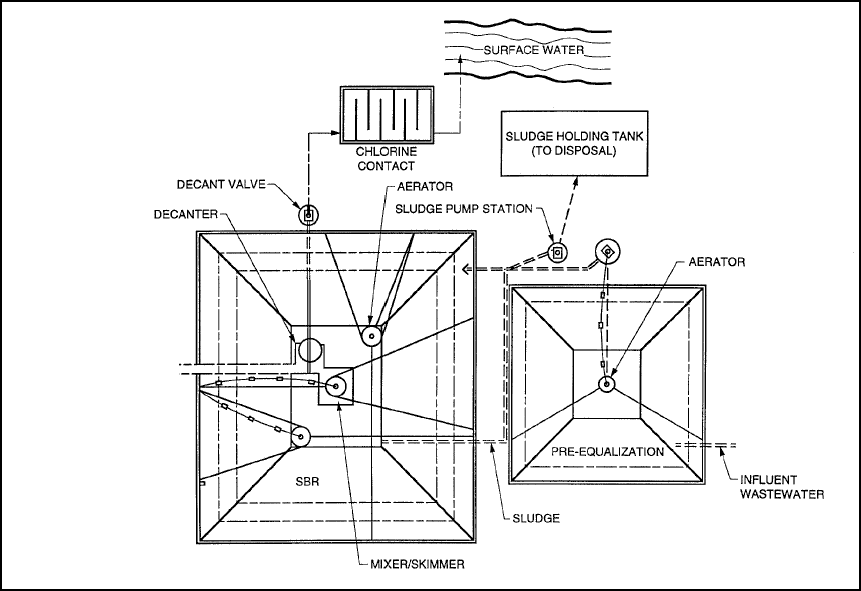
Figure E-4. Sequencing batch reactor.
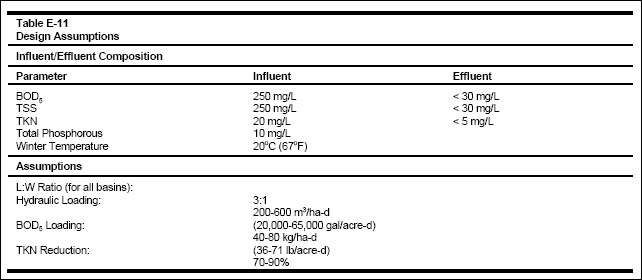
(7) Influent valves: 2, each 150 mm (6 in.) diameter.
(8) Air blower values: 2, each 150 mm (6 in.) diameter.
6. Constructed Wetlands Aerobic Non-Aerated Hyacinth System...
Design an aerobic non-aerated hyacinth constructed wetlands secondary treatment system for a municipal wastewater flow of
284,000 L/d (75,000 gal/d). The system will require preliminary or pretreatment (Imhoff tank). Disinfection of effluent may
be required depending upon regulatory restrictions.
( a ) BOD loading.
Calculate the influent BOD loading using the influent BOD concentration and the design flow, as shown in Table E-12.
( b ) Basin surface area.
Calculate the required basin surface area at moderate BOD loading rate of 50 kg/ha-d and the required area for the primary
or first cells at a BOD loading rate of 100 kg/ha-d, as shown in Table E-12.
( c ) Primary cell dimensions.
Use two primary cells. Calculate the dimemsions of the two primary cells, as shown in Table E-12.
( d ) Final cell dimensions.
Use four final cells. Calculate the dimensions of the four final cells, as shown in Table E-12.
( e ) Cell volume.
Calculate the primary and final cells volume, as shown in Table E-12. Allow 0.5 m (1.6 ft) for sludge storage and assume
1.2 m (4 ft) effective water depth for a total pond depth of 1.7 m. Use a 3:1 sideslopes ratio to determine the treatment
volume (approximation of a frustrum).
( f ) Hydraulic detention time.
Estimate the hydraulic detention time in the effective, or above-sludge, level (zone) in the primary and final cells, as
shown in Table E-12.
( g ) Hydraulic loading.
Check the hydraulic loading to ensure that a minimum of 75 percent total nitrogen reduction is achieved to comply with the
effluent quality requirements, as shown in Table E-12.
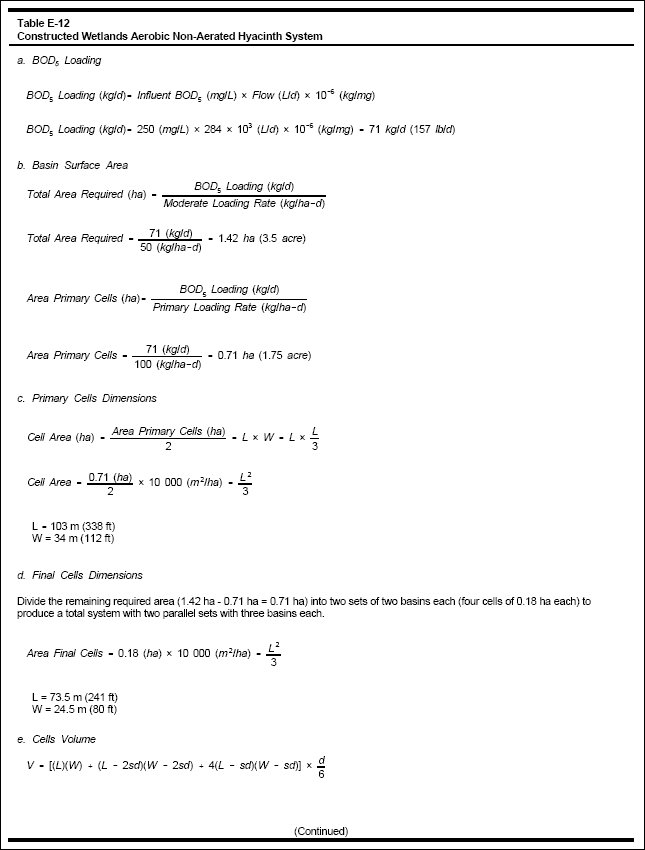